Remotely located wireless devices require extra-long-life batteries to reduce the cost of ownership.
Sol Jacobs • Tadiran Batteries
Remote wireless devices serve as the cornerstone for all types of IIoT-related applications, including asset tracking, system control and data automation (SCADA), environmental monitoring, AI, and machine learning, to name a few.
Applications that are easily accessible and that operate in relatively moderate temperatures typically allow for use of numerous battery technologies, including inexpensive consumer alkaline and lithium-ion rechargeable batteries. However, the choice of power source becomes far more critical for wireless devices in scorching deserts, the frigid Arctic, or other harsh environments. Choice of battery technology is especially important where battery replacement is difficult or impossible, or if the application involves extreme temperature cycling. Specifying the right battery becomes even more important if the application requires 10+ year battery operating life to reduce the cost of ownership.
It is useful to review the properties of ultra-long-life lithium battery chemistries to see how their benefits relate to the real world. There are two types of low-power wireless devices. The vast majority of them draw microamps of current and are powered by industrial-grade primary (non-rechargeable) lithium batteries. The second type of device draws current in the milliamp range. This level is enough to exhaust a primary battery relatively quickly. So a better approach may be an energy harvesting device in combination with an industrial grade rechargeable lthium-ion (Li-ion) cell to store the harvested energy. Low-power devices that require two-way connectivity often utilize a low-power communications protocol (i.e. WirelessHART, ZigBee, LoRa) along with a low-power chipset to maximize battery life.
As the lightest non-gaseous metal, with a high intrinsic negative potential that exceeds all others, lithium offers the highest specific energy (energy per unit weight) and energy density (energy per unit volume) of all commercially available chemistries. Lithium cells operate within a normal operating current voltage (OCV) range of 2.7 to 3.6 V. They are also non-aqueous, making them less likely to freeze in extremely cold temperatures.
Numerous factors must be considered when specifying a battery, including the amount of current consumed during active mode (including the size, duration, and frequency of pulses); energy consumed during ‘stand-by’ mode (the base current); storage time (as normal self-discharge during storage diminishes capacity); thermal environments (including storage and in-field operation); and equipment cut-off voltage, which drops as cell capacity is exhausted or during prolonged exposure to extreme temperatures. Perhaps most critical is the battery’s self-discharge rate, which can exceed the amount of energy consumed to operate the device.
Primary battery chemistries include iron disulfate (LiFeS2), lithium manganese dioxide (LiMNO2), lithium thionyl chloride (LiSOCl2), alkaline, and lithium metal oxide chemistry.
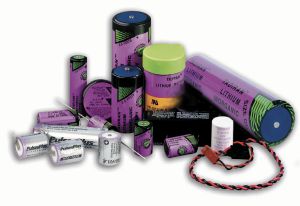
LiSOCl2 chemistry is overwhelmingly preferred for ultra-long-life applications. These cells can be constructed two ways: bobbin-type or spiral wound. Bobbin-type LiSOCl2 batteries feature higher capacity and energy density along with extremely low annual self-discharge, under 1% per year for certain cells that can operate for up to 40 years. Bobbin-type LiSOCl2 cells also feature the widest possible temperature range (-80 to 125°C) and a glass-to-metal hermetic seal to help prevent leakage.
Battery self-discharge varies depending on the method of manufacturing and the purity of raw materials. For example, a superior quality bobbin-type LiSOCl2 cell can feature a self-discharge rate of 0.7% per year, retaining 70% of its original capacity after 40 years. By comparison, a lower quality bobbin-type LiSOCl2 cell can have a self-discharge rate as high as 3% per year, losing 30% of its capacity every 10 years, making 40-year battery life impossible.
Higher self-discharge rates may take years to detect, and theoretical test data is often misleading. So thorough due diligence is necessary when evaluating potential battery suppliers.
High pulses
Remote wireless applications increasingly require periodic high pulses to power two-way wireless communications. Standard bobbin-type LiSOCl2 cells are not designed to deliver high pulses. This can be easily overcome by adding a patented hybrid layer capacitor (HLC). The standard bobbin-type LiSOCl2 cell delivers low daily background current, while the HLC stores energy that can produce pulses of up to 15 A. The HLC also features a unique end-of-life voltage plateau that enables ‘low battery’ status alerts.
Supercapacitors are generally not well suited for industrial applications due to various limitations, including: short-duration power; linear discharge qualities that do not permit full discharge of available energy; low capacity; low energy density; and a high self-discharge rate of up to 60% per year. In addition, supercapacitors linked in series require cell-balancing circuits that boost cost and take up space, use crimped seals that may leak, and draw more energy to shorten their operating life.

Benefits of bobbin-type LiSOCl2 chemistry become clear from a review of remote wireless applications that employ these batteries. In one, researchers are studying water channels up to 2.5-km deep beneath glaciers in Greenland and Antarctica to better understand the effects of climate change on rising sea levels. To support this research, an innovative new instrument, dubbed the Cryoegg, was developed by Cardiff University.
The Cryoegg transmits data wirelessly using radio transmissions, thus eliminating the need for bulky and expensive cables that can be damaged by glacial movement. The product designers were looking for a power source superior to lithium polymer batteries. Instead, they chose a bobbin-type LiSOCl2 cell that delivers high capacity and energy density and, is able to withstand temperatures as low as -30°C. It is capable of delivering periodic high current pulses to transmit data twice daily for approximately two years to continually monitor changes in temperature, pressure, and electrical connectivity.
This application utilizes the 169 MHz Wireless M-Bus radio technology commonly found in AMR/AMI utility meter transmitter units (MTUs). Utility metering applications demand the use of bobbin-type LiSOCL2 batteries to reduce the risk of large-scale battery failure that can disrupt billing systems and disable remote start-up/shut-off capabilities.
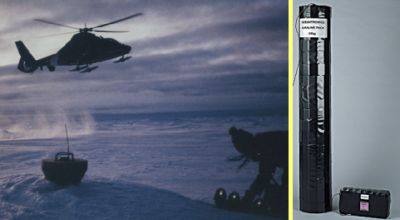
Another application involves the transport of scientific equipment across the Arctic environments. Oceantronics in Honolulu redesigned the battery pack for its GPS/ice buoy, replacing 380 alkaline D cells with a far smaller, lighter, and more economical battery pack using 32 bobbin-type LiSOCl2 cells and four HLCs.
The new battery packs reduce size and weight by 90% (54 kg down to 3.2 kg). The compact size enables Oceantronics to make its device far easier to transport via helicopter to icebergs near the South Pole. Switching from alkaline to LiSOCl2 chemistry also extended the operating life of the device many fold.
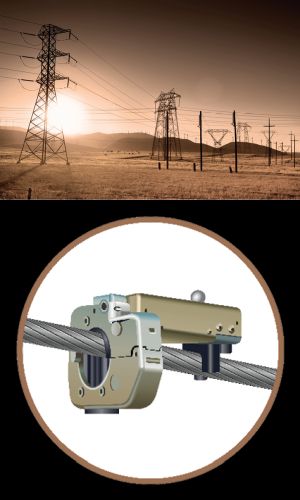
In a third application, LiSOCl2 cells reduced the size and weight of line/connector sensors that monitor the status of electric power transmission lines located in desert environments. Southwire line/connector sensors utilize bobbin-type LLiSOCl2 batteries to power the collection, aggregation, and transmission of data via a cellular network, monitoring temperature, catenary cables, and line current to warn the utility if transmission lines go down.
Use of a bobbin-type LiSOCl2 battery enables these devices to be compact and lightweight (3.5 lb) for easier portability. These batteries can handle an extreme temperature range of -40 to 50°C and provide the high energy density necessary to deliver over 45 days of maintenance-free back-up power if no line current is detected.
Energy harvesting
Energy harvesting is a candidate for applications that draw milliamps of current, enough to prematurely exhaust a primary battery. Photovoltaic (PV) panels are the most proven form of energy harvesting. Energy can also be harvested from equipment movement, vibration, temperature variances, and ambient RF/EM signals.
For example, small solar panels are being combined with industrial grade Li-ion batteries to track the health and status of animal herds. Solar/Li-ion hybrid systems also power parking meter fee collection systems, using AI-enabled sensors to identify open parking spots.
Consumer-grade rechargeable Li-ion cells deliver a maximum operating life of five years and 500 recharge cycles and operate within a moderate temperature range (0 – 40°C), with no ability to deliver high current pulses. By contrast, industrial grade Li-ion batteries can operate for up to 20 years and 10,000 full recharge cycles, with an expanded temperature range (-40 to 85°C), and are able to deliver high pulses to power two-way wireless communications.
In summary, long-life primary and rechargeable lithium batteries can reduce the cost of ownership for remote wireless applications. To ensure that the battery will last as long as the device, you must do your due diligence by requiring prospective battery suppliers provide well-documented long-term test results, in-field performance data under similar environmental conditions, along with numerous customer references.