In many cases, the difference is related to regulatory demands versus environmental demands. Both segments require high levels of safety and performance from Li batteries. Medical applications have numerous strict regulatory and certification requirements while industrial systems tend to have more challenging environmental performance needs.
This FAQ looks at the extensive standards defined for medical batteries, including the use of certified production facilities, then compares medical and industrial Li metal oxide (LMO) primary batteries, Li thionyl chloride (LiSOCl2) primary batteries, and Li-ion rechargeables for industrial, commercial and medical applications. It closes by looking at a possible catholyte to replace the cathode and electrolyte in implanted pacemaker batteries.
Medical standards
ANSI/AAMI ES 60601-1 is the most comprehensive single reference for medical electrical equipment standards including for battery-operated devices. It includes a risk management assessment model, a framework for essential performance, measuring the effect of a device on user and patient safety, and manufacturing methods to demonstrate safety or alternate compliance methods.
ANSI/AAMI ES 60601-1 requires that primary Li batteries comply with IEC 60086-4, primary batteries — part 4: safety of lithium batteries and that rechargeable Li batteries comply with IEC 62133, secondary cells and batteries containing alkaline or other non-acid electrolytes. Both the IEC standards specify requirements and tests for safe battery use under intended operating conditions and foreseeable misuse.
In the US the Federal Drug Administration (FDA) regulates medical products including battery-powered devices. Manufacturers are required to document that products comply with relevant consensus standards or standards recognized by the FDA, including (note that these are not exclusively medical standards and that some apply to consumer, commercial, and industrial batteries, but these are recognized by the FDA):
- IEC 60086-4, Primary Batteries – Part 4: Safety of Lithium Batteries
- IEC 60086-5, Primary Batteries – Part 5: Safety of Batteries with Aqueous Electrolyte
- IEC 62485-X, Safety Requirements for Secondary Batteries and Battery Installations
- IEC 62133, Secondary Cells and Batteries Containing Alkaline or Other Non-acid Electrolytes
- UL 1642, Lithium batteries, covers many applications including pacemakers and electronic medical devices. It includes guidelines that set a limit on the amount of lithium in primary and secondary batteries and individual cells in two use cases:
- If the battery is “technician-replaceable”, the battery can contain a maximum of 5.0 grams of metallic lithium.
- If the battery is “user-replaceable”, each battery can contain a maximum of 4.0 grams of metallic lithium, and each cell should contain a maximum of 1.0 grams of metallic lithium.
- UL 2054, Household and Commercial Batteries
In addition to those battery-specific standards, there are equipment standards that include battery requirements for specific medical procedures and applications:
- ISO 7176-25, Wheelchairs – Part 25: Batteries and Chargers for Powered Wheelchairs.
- ISO 15004-1, Ophthalmic Instruments – Fundamental Requirements and Test Methods – Part 1: General Requirements Applicable to all Ophthalmic Instruments
- ISO 20127, Dentistry – Powered Toothbrushes – General Requirements and Test Methods
Production in a UL-certified facility
Another difference between medical and industrial batteries is that while there are recommendations related to the production of industrial batteries, to comply with the FDA’s medical certifications, Li batteries must be produced in a UL-certified factory. All aspects of battery manufacturing from initial chemistry production to fabricating the cells and assembling the packs must take place in UL-certified facilities. There are significant documentation requirements from UL, IEC, and FDA for medical devices including batteries.
In addition to specific requirements, there are general guidelines for medical device manufacturers to help ensure that products are produced in a manner that minimizes the potential for serious product malfunctions and helps ensure operator and patient safety. These guidelines also include recommendations for supply chain management to, should an error occur, help trace the root cause to then help identify and correct the source of the problem. The supply chain management protocols require documentation from battery makers as well as from device manufacturers.
LMO for medical and industrial uses
Lithium metal oxide (LMO) primary batteries use a carbon-based anode, a non-toxic and non-pressurized electrolyte, and anode materials that are less reactive than standard Li cells. They are available in high-power and medium-power designs for use in medical, military, and industrial applications. LMO batteries have a nominal voltage of 4 V, up to 2 Wh or more of energy, and can support continuous currents up to 5 A and pulses up to 15 A. With a self-discharge rate under 2% per year, these batteries can deliver up to 20-year operating lives. LMO batteries have an operating temperature range of -55 to 85 °C and a hermetic seal for added safety.
LMO batteries are sited for medical applications that need instantly available continuous high-rate power and long operating and shelf lives. Typical applications include (Figure 1):
- automatic external defibrillators
- bone drills and bone growth simulators
- infusion pumps
- surgical power tools
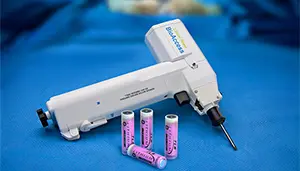
Figure 1. Cordless bone drill powered with LMO batteries (Image: Tadiran).
Thionyl chloride for industrial and medical
Lithium thionyl chloride (LiSOCl2) primary batteries are widely used in industrial applications. These batteries are offered in high-rate spiral constructions and high-capacity bobbin constructions. The combination of high voltage, very low self-discharge rates, operating temperature ranges up to -40 to +85 °C, and hermetically sealed designs make these batteries suited for a range of industrial applications, and bobbin cells can provide operating lifetimes of up to 40 years in some cases. Modified LiSOCl2 bobbin cells are available that can operate at temperatures as low as -80 °C and as high as 125°C, making them suited for specialty industrial and medical applications. For example, batteries rated for -80 °C can be used in medical cold chains to ensure the quality of pharmaceuticals, vaccines, and tissue samples while batteries rated for 125°C can be used in devices that require autoclave sterilization.
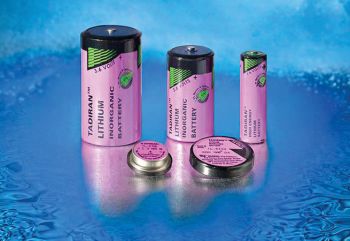
Figure 2. These bobbin-type LiSOCl2 batteries operate down to -80 °C (Image Tadiran).
Industrial and medical Li-ions
Like their primary counterparts, industrial-grade Li rechargeable batteries (Li-ions) address the performance limitations of consumer-grade batteries. Consumer (also known as commercial) Li-ions are limited to 5-year operating lives, 500 to 1,000 recharge cycles, and a high self-discharge rate of up to 60% per year. In addition, commercial cells have a limited operating temperature range of 0 to 60 °C, can’t support high current pulses, and cannot be recharged at very low temperatures. Industrial Li-ions address all those limitations:
- operating life up to 20 years
- up to 5,000 full charge cycles to 100% depth of discharge
- -40 to 85 °C operation and charging temperature range and storage up to 90 °C
- high current pulses (up to 5A for AA cell)
- less than 5% per year self-discharge
- special designs are available that can withstand autoclave sterilization for medical applications
As an example of an application for these batteries, industrial Li-ion energy systems are available in standard formats that can replace lead-acid batteries in existing lift truck designs (Figure 3). Standard lift truck Li-ion packs are available that deliver 24, 48, and 80 V. They are available with charging systems designed for use in industrial environments. And some designs are modular enabling users to dial in the amount of energy storage demanded by specific application cases or different vehicle types.
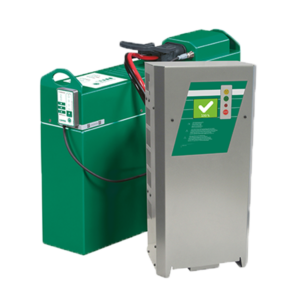
Figure 3. Li-ion battery packs are available in standard sizes to replace lead-acid batteries in industrial lift trucks (Image: HOPPECKE Batterien).
Better implantable batteries
Researchers have developed a possible way to improve the energy density of primary batteries that could enable a 50% increase in operating life while also decreasing weight and size. Primary batteries are already preferred in implanted applications like pacemakers since they offer about three-times the energy density of rechargeable batteries. Higher energy density means longer operating life and fewer procedures to replace drained batteries. The new chemistry also works well at human body internal temperature, a key consideration.
Today’s pacemaker batteries have a typical lifetime of 5 to 10 years. The new chemistry could last up to 30 years in some cases. The development of a new type of electrolyte is the key to higher performance. In a typical battery, the electrolyte does not contribute to energy storage density. The researchers developed a fluorinated liquid compound that combines some of the functions of the electrolyte and cathode. The new material is called a catholyte and supports a significant weight reduction. Additionally, the catholyte material is compatible with the Li carbon monofluoride (LiCFx) chemistry used in current pacemaker batteries. As the battery is discharged, the catholyte changes its chemical composition (Figure 4).
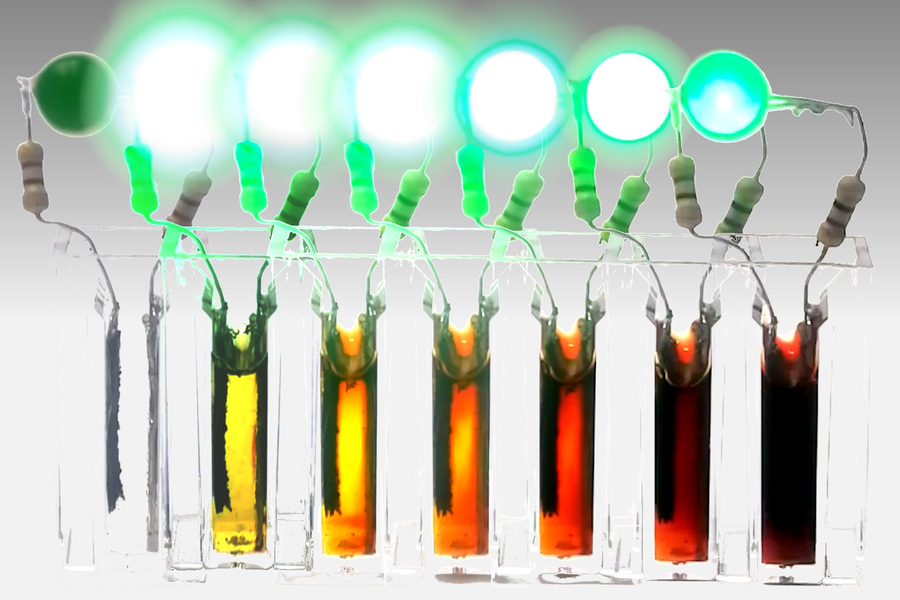
Figure 4. Time-lapse images from left to right show the catholyte material chemically changing and turning darker and darker red as the battery discharges (Image: MIT).
The new catholyte material is also compatible with existing battery manufacturing processes for pacemaker batteries. The catholyte material is similar in cost to the material it’s replacing meaning that the long-life batteries should be comparable in cost to today’s designs. There’s still work to be done to finalize the testing and validation of the catholyte material. It could find its way into pacemaker battery development and qualification in a year or two. In the future, if its operating temperature range can be extended, the catholyte-based batteries may provide an alternative to LiSOCl2 in low-rate industrial applications that require 20 to 40-year operating lives.
Summary
Industrial and medical applications rely on many of the same Li battery chemistries. The differences lie in the safety standards demanded for medical batteries and devices, and the environmental expectations for industrial batteries. Niche applications in both the industrial and medical areas require extreme temperature operation from Li batteries. New materials are under development to enable the production of higher-density batteries for implantable applications like pacemakers.
References
How to choose the Best Industrial Lithium Battery, Medatech
Making Sense of Regulations for Medical Device Batteries, CSA Group
Medical Batteries, Tadiran
New materials could enable longer-lasting implantable batteries, MIT
Tell Us What You Think!